January 11, 2013 — In the decades since the publication of Rachel Carson’s environmental classic Silent Spring, since the incidents of pollution that caused the Cuyahoga River to catch fire in 1969 and contaminated residents of Love Canal in the 1970s, our knowledge of how synthetic chemicals—chemicals that are made in laboratories but not found in nature—make their way into the environment and how they interact with living cells has grown remarkably.
We now know that many such chemicals enter the environment, not only from smokestacks, drainpipes, leaky storage tanks and waste sites, but also as they migrate from furniture, textiles, building materials, electronics, toys, personal care products, packaging and many more manufactured goods we encounter every day. As a result, many of these chemicals are present in indoor air and dust. Many are traveling the global environment with air and ocean currents. Many are in the food web and in our bodies.
Almost every week, new scientific studies are published documenting adverse health effects of synthetic chemicals.
At the same time our understanding of the sources of chemical exposure has been expanding, so has our knowledge of how chemicals behave biologically. Since well before the publication of Silent Spring, scientists have been aware of the potential adverse environmental and health effects of industrial chemicals. Attention to these impacts typically focused on acute and immediate effects resulting from high levels of exposure. But we now know that many widely used synthetic chemicals can interact with living cells at very low levels of exposure in ways that produce profound effects on development, metabolism, neurological function, reproduction and other vital body systems, sometimes affecting more than one generation. Almost every week, new scientific studies are published documenting adverse health effects of synthetic chemicals such as bisphenol A, brominated flame retardants, phthalates, persistent pollutants or endocrine disruptors—chemicals most of us encounter daily.
The discovery that our lives are filled with so many potential sources of exposure to chemicals with so many subtle but significant impacts has prompted the need for a pollution prevention strategy that goes well beyond putting filters and scrubbers on chimneys or treating wastewater. It has catalyzed the creation of a new approach to designing molecules that aims to prevent problems from occurring in the first place: green chemistry.
Radical Departure
The most fundamental principle of green chemistry is that the best way to prevent harmful chemical pollution is to design materials that are inherently environmentally benign and safe for human health. Green chemistry works toward this goal by using resources efficiently, eliminating use of inherently toxic ingredients and chemical combinations, eliminating waste and hazardous by-products, and minimizing use of energy throughout a product’s entire life cycle.
While this seems like common sense, it represents a radical departure from the status quo.
Asking synthetic chemists—scientists in the business of creating new molecules—to think about a molecule’s biological and ecological behavior and its environmental footprint adds an entirely new dimension to their work. Historically, such considerations have been absent from synthetic chemistry. Chemists are not required to have any formal training in toxicology or other environmental health science that would enable them to understand ecological impacts at the molecular level. John Warner, president and chief technology officer at the Warner Babcock Institute for Green Chemistry, has said of his early career as a commercial chemist, “I have synthesized over 2,500 compounds but have never been taught what makes a chemical toxic.”
Understanding what makes a chemical product safe is the challenge of green chemistry.
Warner and Paul Anastas, assistant administrator for the U.S. Environmental Protection Agency’s Office of Research and Development, are widely regarded as the founders of green chemistry. In their book, Green Chemistry Theory and Practice, Anastas and Warner outlined 12 principles of green chemistry, guidelines for working chemists to consider as they set out to design new compounds to minimize—and ideally eliminate—the risk of creating molecules that will threaten the health of humans or the environment.
But Warner points out these principles are just the start. “We have to realize [that bringing green chemistry into practice] is an endless process,” he says.
Understanding what makes a chemical product safe is the challenge of green chemistry, Lynn Goldman, dean of George Washington University School of Public Health and Health Services, told attendees at the American Chemistry Society’s 15th Annual Green Chemistry & Engineering Conference in Washington, D.C., in June.
Knowledge of how chemicals behave has grown well beyond that on which our current system of regulating chemicals was based, Goldman explained. To understand what makes a molecule safe or toxic, we now have to take into account endocrine active compounds, how environmental exposure to chemicals can alter how genes behave, and the many ways in which chemicals can interact with the various dynamic parts of living cells. To put this knowledge into practice, Goldman said, will require “new collaborations between clinicians, chemists, engineers and biologists.”
Firmly Established
Since it was introduced almost 20 years ago, green chemistry has become firmly established as an approach to designing new chemical products and manufacturing processes in ways that make them less hazardous to human health and the environment.
EPA started a green chemistry program in the 1990s that supports research aimed at developing and promoting pollution prevention through the design and synthesis of nontoxic, resource efficient materials. For the past 16 years the agency has awarded Presidential Green Chemistry Challenge Awards to honor “outstanding examples of green chemistry.” These have included new ways to synthesize ibuprofen, bio-based plastics, nontoxic adhesives, water-based high-performance paint and non-toxic chemical cleaning agents that can neutralize persistent toxics.
EPA’s Design for the Environment Program is using green chemistry to support work to develop nontoxic commercial cleaning products, to assess alternatives to hazardous flame retardants and to find safe replacements for products based on bisphenol A. Green chemistry is also part of numerous individual state policies aimed at preventing exposure to hazardous chemicals, among them efforts in California, Maine, Massachusetts, Michigan and Washington. In Europe, chemicals management policies—among them the European Union directive known as REACH (Registration, Evaluation, Authorization and Restriction of Chemical Substances)—are providing incentives for green chemistry innovation, and green chemistry education is being incorporated into university-level curricula in China and India.
“The concept of sustainable chemistry is a great framework for understanding how to approach things.” — Rich Helling, Dow Chemical associate director of sustainability
In the United States, efforts are underway to improve chemists’ ability to evaluate synthetic chemicals based on the many ways they can interact with living cells. Adelina Voutchkova of the Yale University Center for Green Chemistry and Green Engineering presented one example of such an evaluation tool at the ACS conference. Also at the conference, Raymond Tice, chief of the U.S. National Toxicology Program’s Biomolecular Screening Branch, presented the new toxicology tools being developed by the NTP and National Institute of Environmental Health Sciences that will enable scientists in academia and regulatory agencies to evaluate chemicals for the wide range of behaviors green chemistry considerations demand.
Rich Helling, associate director of sustainability/life cycle assessment at Dow Chemical, says his company is training its research and development scientists to do “early screening” of new products and to consider materials from a perspective of “atom and energy efficiency, hazard reduction, and holistic design,” and thus “pick more sustainable projects.”
Green chemistry efforts at Dow have gone into producing a number of industrial chemicals, among them a bio-based plasticizer, explains Helling. They have enabled Dow to meet customers’ requests for products that do not contain “particular chemicals of concern, such as phthalates and lead,” says Helling. They have also gone into Dow’s production of a new compound to replace a flame retardant that has been discovered to be environmentally persistent and environmentally mobile. And in August Dow for the first time publicly presented its new research and development sustainability footprint tool that is being applied throughout the company’s R&D efforts.
“The concept of sustainable chemistry is a great framework for understanding how to approach things,” says Helling. Improving the safety profile of a new process or product is “good process chemistry,” he explains. Any process that is not less hazardous or less complex “slows down your research,” he says.
Next: Education
“The case for green chemistry has been made,” says Amy Cannon, executive director and co-founder with Warner of Beyond Benign, a nonprofit organization devoted to green chemistry education. “What is next? A more systematic approach that’s really going to change the way we educate scientists.”
Teaching future chemists what makes a molecule toxic is essential to advancing green chemistry, agrees Warner. “Without this we can’t accomplish our goals.”
Green chemistry advocates are approaching this at the professional level, fostering working partnerships between environmental health scientists and synthetic chemists through efforts such as the collaborations being facilitated by the nonprofit Advancing Green Chemistry. They’re also pushing for change in education, creating new curricula for students beginning at the K–12 level and extending through and beyond college and university education.
Warner underscores that simply showing students and professional chemists examples of green chemistry products and asking them to learn the principles of green chemistry is not sufficient, however.
“It would be like showing examples of Russian poetry—an alliteration, an allusion, a time juxtaposition, all in Russian—to people who don’t speak Russian and then asking them to write a Russian poem,” he says. What’s needed is an understanding of what makes molecules behave—how their chemical composition and structure influences their biological and physical behavior. Creating a molecule to perform a certain task (the traditional goal of synthetic chemistry) without considering the full range of its potential ecological interactions falls short of green chemistry’s goals.
Creating a molecule to perform a certain task (the traditional goal of synthetic chemistry) without considering the full range of its potential ecological interactions falls short of green chemistry’s goals.
Marc Hillmyer and William Tollman are preparing chemistry students for careers in which green chemistry will be a given rather than a novelty. In 2010–11 the University of Minnesota faculty taught a three-credit course in green chemistry for chemistry majors. This information, Hillmyer says, is essential to training the next generation of scientists to create new materials that “don’t have negative health consequences, will reduce our reliance on petroleum” and have what Hillmyer calls “programmed end-of-life designs.” By this he means making new synthetic chemicals that are designed “in environmentally friendly ways to be recycled or reconstituted.” Hillmyer, whose specialty is polymers, points out that it’s important to “think about this at the front end” rather than after the fact—as shown by the impacts of countless extremely useful but hazardous chemicals.
Like Cannon and Warner, Hillmyer acknowledges that we don’t yet have all the tools—educational or toxicological—that are needed.
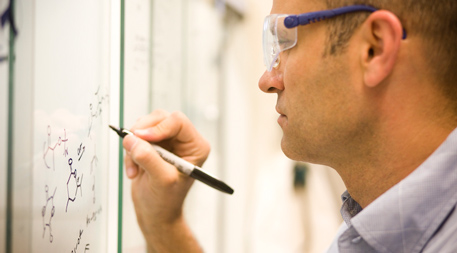
Chemistry professor Marc Hillmyer directs the Center for Sustainable Polymers at the University of Minnesota. Photo by Josh Kohanek.
“This is a great area for growth,” he says. Filling this gap was part of the impetus for developing a green chemistry course that would be part of the general chemistry curriculum.
“We made a conscious choice to teach this to our chemistry majors,” he explains. “We need to get this into the minds of people who are going to work for companies like Dow and DuPont.”
Students seem to need no persuasion: “Our course was oversubscribed. We had to turn people away,” says Hillmyer.
This fall, Cannon and Warner are teaching a course at Simmons College that Cannon says is “most likely the first toxicology course to be required of chemistry majors.” Warner explains that what this class will teach is “mechanistic toxicology,” which will begin to teach students “how to look at a molecule and know if it’s toxic.”
Warner uses the term “toxic” broadly, to include molecules that bind to DNA, are greenhouse gases or cause ozone depletion.
“If I could wave a magic wand, mechanistic toxicology would be the moral responsibility of anyone making a material,” Warner says.
Clearly there are no magic wands. But the challenging process of what Anastas describes as “changing how we define goals and performance” to produce new molecules that are more environmentally benign, more economically viable, and that will rival or outperform existing materials, is clearly underway.
This feature originally appeared in the Fall 2011 issue of Momentum magazine, Ensia’s predecessor, as “Chemistry Goes Green.”
Ensia shares solutions-focused stories free of charge through our online magazine and partner media. That means audiences around the world have ready access to stories that can — and do — help them shape a better future. If you value our work, please show your support today.
Yes, I'll support Ensia!
Kindly Check our Website: https://www.meetingsint.com/conferences/green-chemistry
Regards,
John Brown
Email Us: greenchem@insightsummits.com
Call/WhatsApp +65 3158 1626